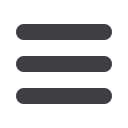
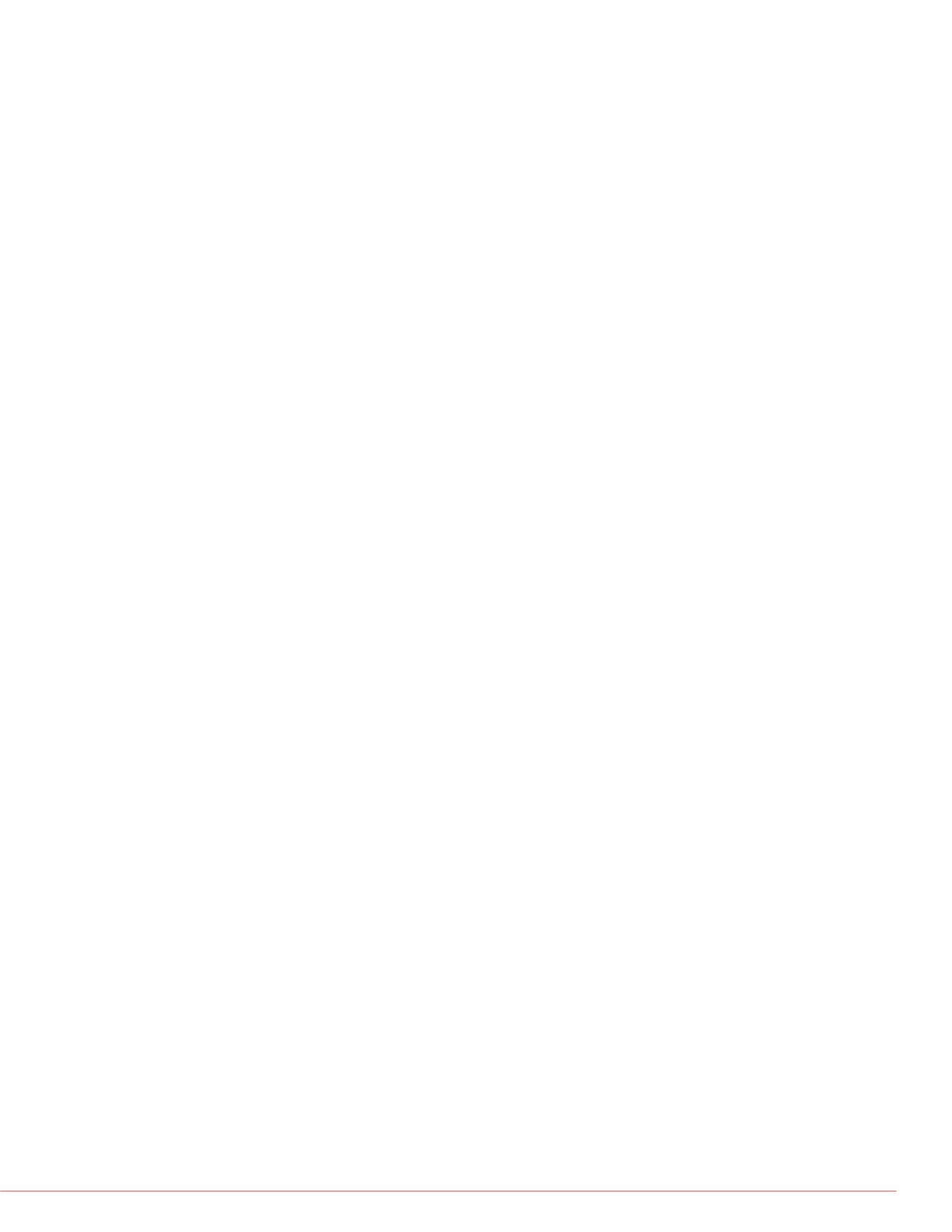
3
Thermo Scienti c Poster Note
•
PN ASMS13_T597_JBeck_E 07/13S
of ultrahigh-performance liquid
nal parameters of a UHPLC-
lysis of quaternary ammonium
bile phases of different pH
of PQ and DQ on a Thermo
specifically designed for this
changes of mass spectral
chromatograms (XIC) obtained
ing potential (corona voltage) of
ased on results obtained from
us identification of PQ and DQ
er analytical data with superior
e, C
12
H
14
N
2
Cl
2
) and diquat (DQ,
are quaternary amines widely
for both terrestrial and aquatic
d/or ingestion. The Ontario
169/03) has a standard of 70
uat is also regulated by the
(EPA) at a maximum
hile PQ is unregulated by the
CL of 0.1 µg/L for any individual
es. Different data quality
te the need for a
sitivity (i.e. <0.1 µg/L or better)
de the separation by ion-pairing
philic interaction liquid
ng either ultraviolet (UV) or mass
gy, method detection limits
nd high ng/L for DQ. A 2012 U.S.
nd 150,000 pounds of PQ and
C-MS/MS) using an ESI
Q analysis since late 1990s.
rce used, the deprotonated
the singly charged radical ion
ss extent, the doubly charged
PQ) have been observed in the
RM) transitions used in the
ile phase. Commonly used
and deprotonated cation [M –
charged quasi molecular ion M
2+
M transitions for PQ and DQ
asses 15 ([M – CH
3
]
+
,
m/z
170)
at
m/z
168 ([(M – H) – CH
3
]
+
)
3). Product ions resulted from
or 42 ([(M – H) – CH
3
– HCN]
+
,
N]
+
) for DQ analysis (Ref. 4). A
transitions may be used in the
3, 185 and 186) and DQ (
m/z
ferentiated by 1 amu. As the DQ
– H]
+
of PQ, one might expect
LC separation and MS data
nown to have high ionization
spectral peak of DQ contributing
ts obtained for PQ might be
etween pH of mobile phase and
ns of PQ DQ, the root cause of
rbitrap MS method for the
of different jurisdictions.
Methods
Sample Preparation and Chemicals
Individual stock solutions of PQ and DQ were purchased from Ultra Scientific
Analytical Solutions (Brockville, ON, Canada). Neat standards of deuterium (D)
labelled PQ (D
8
-PQ) and DQ (D
4
-DQ) were purchased from CDN Isotope (Pointe-
Claire, QC, Canada). Native and D-labelled intermediate standard solutions were
prepared by mixing the corresponding DQ and PQ stock solutions. Five levels of
analytical standard solutions were prepared by diluting intermediate solutions with
nanopure water (pure water, generated by passing reverse osmosis water through a
Thermo Scientific™ Barnstead™ Nanopure™ water purification system, Mississauga,
ON, Canada). Due to the high ionic strength of PQ and DQ, plastic labware and/or
silanized glassware were used to avoid their adsorption onto the glass surfaces.
ACS reagent grade ammonium acetate (CH
3
COONH
4
), acetic acid (CH
3
COOH) and
hydrochloric acid (HCl) were purchased from Sigma-Aldrich (Oakville, ON, Canada).
HPLC grade acetonitrile (CH
3
CN) was purchased from Fisher Scientific (Ottawa, ON,
Canada). The current method employs direct injection that does not requires sample
preparation. Environmental samples were collected in a 500 mL polypropylene bottle
and refrigerated at 5 3 ºC until analysis. Drinking water samples were analyzed as is
while surface water samples were filtered through a 0.2
µ
filter prior to analysis. A 1 mL
aliquot of each sample was transferred to a 1.8-mL plastic autosampler vial, spiked
with 10
µ
L of 500
µ
g/L, D-labelled internal standards to the concentration of 5 ng/mL,
vortexed and stored under refrigeration until analysis.
Ultra High Performance Liquid Chromatography
The Thermo Scientific™ Dionex™ UltiMate
TM
3000 UHPLC used in the analysis
consisted of a HRG-3400RS binary pump, WPS-3000 autosampler, and a TCC-3400
column compartment. Separation was achieved on a mixed-mode column Acclaim
Trinity Q1 column (2.1 × 50 mm, 3
μm
), using isocratic elution and mobile phase of
acetonitrile:100 mM, pH5.0 ammonium acetate = 75:25 v/v, at a flow rate 0.45 mL/min.
The column oven was set at 35ºC. Both PQ and DQ were eluted within 5 minutes.
Mobile phases used in the pH effect study were the same composition used in the
analysis but prepared at pH of 3.5, 5, 6.2 and 7.3. Flow injection analysis was done by
using 0.013 mm i.d. x 100 cm polyetherether ketone tubing at a flow rate of 0.4 mL/min
and four different pH levels to determine the pH and declustering potential used in the
UHPLC Orbitrap MS analysis.
Mass Spectrometry
The UHPLC was interfaced to an Thermo Scientific™ Exactive™ Plus Orbitrap MS
using a HESI II probe interface. The Orbitrap MS system was tuned and calibrated in
positive mode by infusion of standard mixtures of MSCAL5. High purity nitrogen
(>99%) was used in the ESI source (35 L/min) as well as in a higher energy collisional
dissociation (HCD) cell, enabling collision induced dissociation (CID) experiment
without precursor ion selection, i.e. “all-ion fragmentation” (AIF). The AIF experiment
was done by using normalized collision energy (NCE) of 35 14 eV. The UHPLC flow
rate of 0.45 mL/min and column used resulted in chromatographic FWHM of 6-8
seconds. Mass spectrometric data were collected using a spray voltage (SV, the
equivalent of declustering potential) of 1700 V, an Orbitrap MS resolving power of
140,000 (defined by the full-width-at-half-maximum peak width at
m/z
200, R
FWHM
),
resulting a scanning rate of > 1.5 scans/sec when using automatic gain control and a
C-trap inject time of 50 msec. Therefore, at least nine data points were available to
accurately define each XIC chromatogram from the UHPLC separation of PQ and DQ.
The effect of SV on the formation of the three different quasi molecular ions of PQ and
DQ was also studied by different SV from 700 to 3200 V.
Data Analysis
Analytical data collected were processed offline using Thermo Scientific™ Xcalibur™,
ExactFinder™ and TraceFinder™ data processing packages depending on the need.
Xcalibur was used to process mass spectral data for graphic presentation. ExactFinder
and TraceFinder softwares were used to derive quantitative data. Depending on the
data, a mass extraction window (MEW) of 5 to 20 ppm (part-per-million) from both
sides of the base peak were used to create XIC and quantitative analysis. Results
were exported to Microsoft
®
Excel
®
for data compilation and statistical evaluation.
Results
Flow Injection Analysis
Figure 1 shows results from the flo
phases of three different pH values
from 3200 to 700 volts, in decreasi
experiment was to determine an op
(SNR) of PQ and DQ measurement
were minimal for PQ and DQ at pH
that DP had very little effect on the
DP of 2000 volts is used throughou
FIGURE 1. Results of flow injecti
Effect of mobile phase pH on the
Table 1 lists accurate mass of the t
(i.e., molecular ion M
2+
, deprotonat
[M]
+
.
), along with their respective
13
of PQ and DQ can be achieved by
respective (M+1) peak and fragme
M
2+
M
2+
(M+1)
Diquat
92.04948 92.55117
Paraquat
93.05730 93.55900
Figure 2 shows mass spectral peak
and C (measured); DQ ([M]
+ .
(M+1)
(M+1)), D (simulated) and F (meas
as well as DQ ([M
2+
- H
+
]
+
) and DQ
and measured (I), as an example. I
delivers excellent mass accuracy m
theoretically simulated ones (Figure
better ESI ionization efficiency than
the use of high resolution MS and a
in the MS domain becomes imperat
TABLE 1. Expected
m/z
of PQ an
Table 2 shows average area counts
obtained from the LC analysis of P
values (i.e., 5, 6.2 and 7.3) and dec
of this experiment was to determine
the LC separation of PQ and DQ.