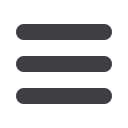
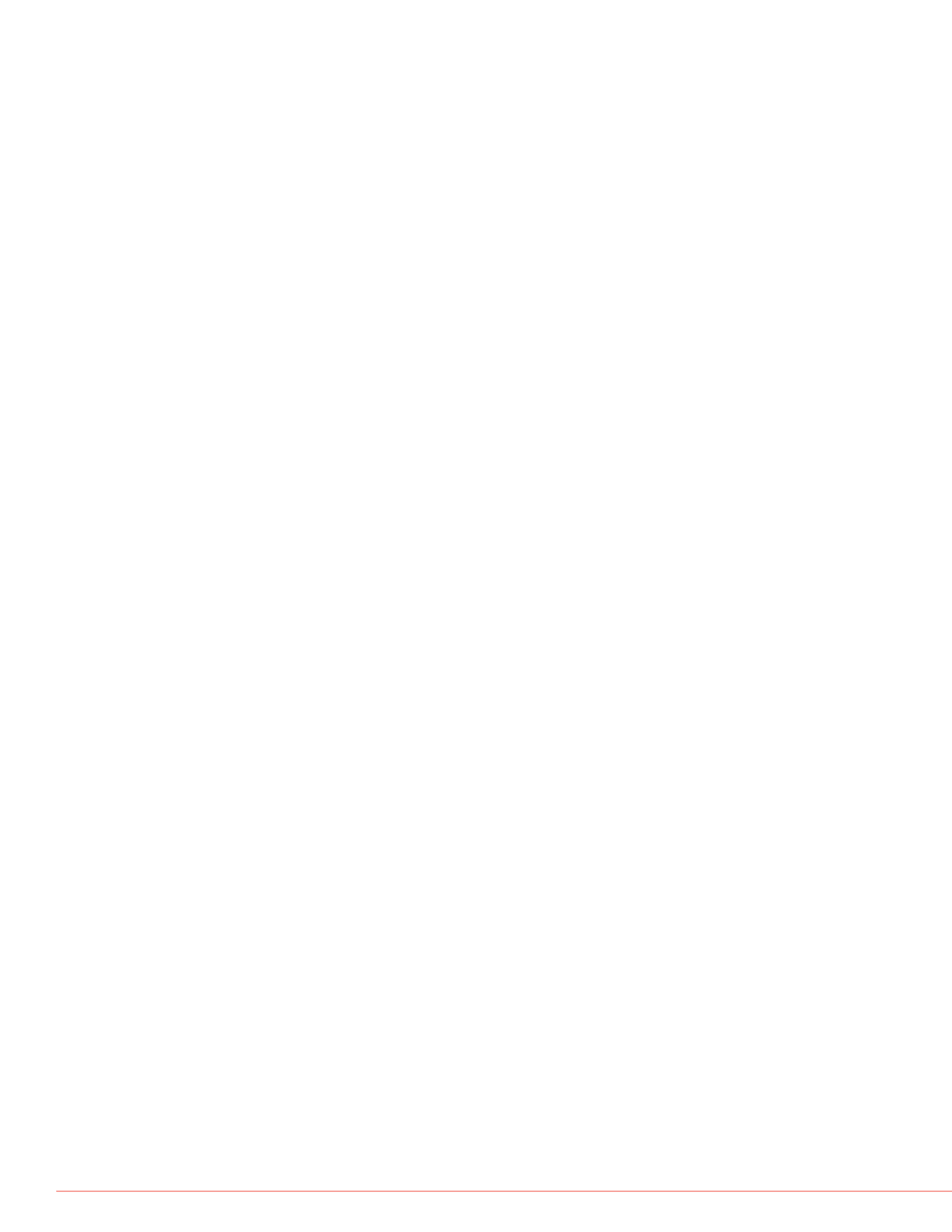
2
Detection and Quantitation of Brominated and Chlorinated Hydrocarbons by DART with Linear Ion Trap and Triple Quadrupole Technology
Overview
Purpose:
Halogenated compounds such as brominated flame retardants (BFRs) and
chlorinated pesticides (OCs) have been in use for many years. Both BFRs and OCs
are persistent in the environment
1
and pose potential health risks. Therefore, detection
and monitoring of these compounds is critical. This experiment is developed to
quantitate BFRs and OCs using liquid chromatography-mass spectrometry (LC-MS).
Methods:
The DART-SVP source (IonSense Corp.) was used to reduce sample
preparation and provide ionization. Both ion trap and triple stage quadrupole (TSQ)
technology were used for this study.
Results:
Ionization modes and fragmentation determined on the linear ion trap were
confirmed on the TSQ. Further optimization and breakdown curves for the TSQ method
were achieved using DART-infusion of the BFRs chosen for further study.
Introduction
Brominated hydrocarbons also known as BFRs have been used in various industries
for decades. Recently, several classes of BFRs have been detected in the biosphere.
OCs have also been used for many years primarily as pesticides, the most infamous of
these being DDT. While most OCs have been banned in the United States, their use
still occurs in developing countries. The continued use of BFRs and OCs, as well as
their persistence in the environment and potential deleterious activity therein, makes
the detection and monitoring of these compounds an important topic. We propose
DART as a simple, rapid, easy-to-use technique; eliminating the need for
chromatographic method development, and reducing or eliminating sample
preparation, for detection and quantitation of both BFRs and OCs.
Methods
Sample Preparation
Compounds listed in Table 1 were dissolved in acetone at 1 mg/mL to make stock
solutions. Stock solutions were diluted serially to give the following standards:
100 ppm, 50 ppm, 5 ppm, 1 ppm, 500 ppb, 100 ppb, 50 ppb, 10 ppb. Kepone was
spiked in at a constant level of 100 ppb as a reference point. Spiked and un-spiked
water samples were analyzed directly with no additional preparation.
DART Methodology
Preliminary data was acquired on the Thermo Scientific LTQ linear ion trap mass
spectrometer using the DART-SVP source in 1D transmission mode, with a grid voltage
of 300V and temperature of 200 ºC. Full scan and MS/MS data were acquired for all
compounds. To confirm the linear ion trap data, further optimize ionization, and obtain
collision energies (CE) breakdown curves, the DART-SVP source was run in direct
infusion mode on the Thermo Scientific TSQ Quantum Access MAX triple stage
quadrupole mass spectrometer. Subsequent quantitation data on the TSQ Quantum
Access MAX™ MS was obtained with the DART-SVP source in 1D transmission mode,
with a grid voltage of 300V and temperature of 400 ºC.
Mass Spectrometry
Negative ion full scan and MS/MS mass spectral data was acquired on the LTQ™
linear ion trap MS with the following conditions: capillary temperature 270 ºC, tube lens
-100V. Negative mode selective ion monitoring (SIM) and selected reaction monitoring
(SRM) were acquired on the TSQ Quantum Access MAX MS with the following
conditions: capillary temperature 200 ºC, skimmer offset 0V. SRM data was acquired
with a Q1 and Q3 resolution of 0.7 FWHM, collision gas pressure of 1.5, with
compound dependent CE and tube lens voltages.
Results
Compound optimization
Initial studies were performed on the linear ion trap MS due to the full scan sensitivity
and high scan rate which is necessary when optimizing on spots with an average
signal duration of 5 to 10 seconds that results when using the DART-SVP in 1D
transmission mode. All but three of the selected compounds were detected and
precursor masses were determined (see Table 1). Additionally, MS/MS spectra were
acquired to determine potential fragments for quantitation (see Figure 2). Confirmation
of the precursor masses was achieved on the TSQ MS using the DART-SVP in direct
infusion mode.
FIGURE 1. Caption is Arial 13 pt Bol
the figure. Figures no longer have a
least one line of space between the l
Always leave space between the fig
Do not change the width of the capti
by side.
Figures spanning multiple
over a foot wide when printed full si
needs to be more than two feet wide
time to read all that detail anyway.
FIGURE 5. Caption.
Compound
Molecular Structure
allyl 2,4,6-tribromophenyl
ether*
1,2,5,6-tetrabromo
cyclooctane*
2,3,4,5,6-
pentabromoethylbenzene
2-bromo-1,3-
bis(dibromomethyl)benzene
hexabromobenzene
tetrabromobisphenol A
tris(2,3-
dibromopropyl)isocyanurate
tetrabrom phthalic
anhydride*
1,2,5,6,9,10-
hexabromocyclododecane
kepone
TABLE 1. Compounds analyzed with
mechanisms, observed precursors, a
masses detected by the linear ion tra
quadrupole with DART-SVP infusion.
not detected initially but were seen wi
Direct infusion was achieved by conne
syringe pump. The needle was held by
was then positioned directly between th
interfaced with the mass spectrometer.
1 to 5 µL/min and a concentration of 10
compounds required higher DART-SVP
than were initially utilized. The optimum
results of the infusion studies shown in
also shows it was possible to ionize the
observed on the linear ion trap MS due
low.
It is interesting to note that the results s
ionization pathway of the molecules. C
non-aromatic carbon, such as tetrabro
the [M-H]
-
species. Alternatively, comp
hydrogen bonded to an aromatic carbo
In addition to optimizing precursor dete
to determine
:
tube lens values, fragme
quantitative experiments on the TSQ M
breakdown curves it was noted that the
linear ion trap, as shown in Figure 2. T
TSQ MS is more energetic than that in