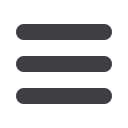
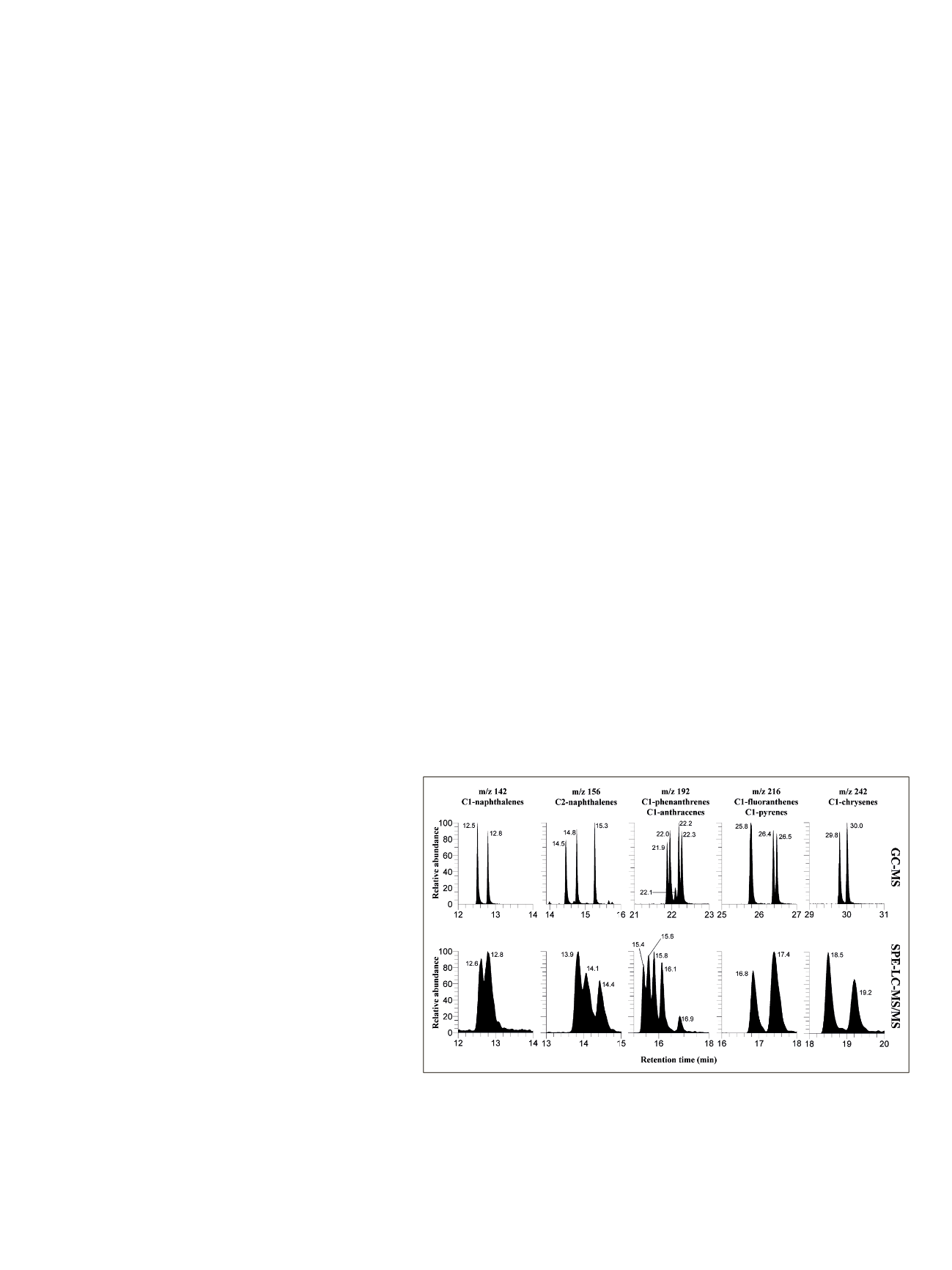
5
Data Analysis
Data analysis was performed using Thermo Scientific
™
TraceFinder
™
EFS software version 3.0.
Results and Discussion
Optimization of Dopant-Assisted APPI Detection
Pure chlorobenzene provides efficient charge transfer
ionization for all PAH in the presence of water, methanol,
and acetonitrile.
19
Therefore, commercially available
high-purity chlorobenzene was used as dopant in this
study without any treatment. Under these conditions, a
strong positive molecular ion (M
+·
) for each analyte was
always observed and isolated as the precursor ion for the
SRM scan events, which is consistent with observations
by other authors who have used chlorobenzene as dopant
for APPI-LC-MS analysis of PAHs.
20
Two programmable syringe pumps and a spraying device
placed in the auxiliary nitrogen gas stream were used.
With this system, little or no backpressure was applied to
the syringe pumps, which translated into stable dopant
delivery. Since analytical signals maximized at a dopant
flow rate of approximately 10% of the eluent flow rate,
using a programmable dopant system has the advantage
of maintaining this optimum ratio as the eluent flow rate
changes during the chromatographic separation.
The spraying system was tested with two syringe pumps
equipped with four 10 mL syringes (40 mL total), which
provided 26 runs (approximately 12 h of continuous
operation) before syringe refills were required. This
translates into a consumption of about 1.5 mL of
chlorobenzene per sample. In comparison, the traditional
LLE-GC-MS approach may require up to 150 mL
(3 × 50 mL extractions) with organic solvents, such as
methylene chloride, to ensure a high recovery.
Chlorobenzene has a much shorter atmospheric persistence
(half-life of 20–40 h) than methylene chloride and is not
considered a carcinogen. Thus, both the lower quantity and
the nature of the halogenated waste produced suggest that
the online SPE-LC-APPI-MS/MS is a more environmentally
friendly methodology than LLE-GC-MS.
Optimization of Chromatographic Separation
During compound optimization for SRM detection, it was
observed that PAHs with the same parent masses have
similar behavior upon collision-induced dissociation (same
product ions, same collision energy, see Table 1), eliminating
the possibility of selective detection of isobaric PAHs.
Because comprehensive PAH analysis requires quantitation
beyond the 16 priority PAHs, a carefully controlled LC
separation is required to solve most of these isobaric
interferences. In addition, since PAH molecules have fixed
planar conformations, chromatographic selectivity is
governed solely by their molecular dimensions.
21
Furthermore, complete chromatographic resolution of the
16 PAHs listed as priority by the EPA using the Hypersil
Green PAH stationary phase has been previously
reported.
22,23
This stationary phase was selected to explore
the possibility of a liquid chromatography separation of
most alkylated PAHs as these compounds are often used
as markers to identify pollution sources and
environmental transformations.
4, 24
Light PAHs
(i.e., alkylnaphthalenes) could be only efficiently separated
using a methanol/water gradient system, as the use of
acetonitrile/water caused fast elution with no resolution
control. On the other hand, methanol proved to be a
weak solvent for PAHs
m/z
228 and above, causing
excessively high retention times and peak shape
broadening even at 100% methanol isocratic elution. A
second gradient between methanol and acetonitrile was
then used after the water/methanol system. Still, retention
times for PAHs
m/z
252 and above were also very high
even at 100% acetonitrile conditions. To perform an
efficient, wide mass range separation, a flow rate gradient
was also used in combination with solvent strength
control, taking advantage of the steep backpressure drop
observed as water is removed from the analytical column
during the gradient.
Figure 2 compares the obtained resolution of alkylated
PAHs contained in the Standard Reference Material 1491a
to that obtained by traditional GC-MS analysis. Although
resolution for C1-naphthalenes was lower than GC, two
marginally resolved peaks are observed in the SPE-LC-MS/MS
separation of these compounds that differ only in the
position of a single methyl group between adjacent carbon
atoms. Since C1-naphthalenes are detected as a group, the
limited resolution does not affect quantitation. As analyte
mass increased, the observed resolution behavior tended to
be similar to that obtained by GC-MS. Both techniques
had the same difficulty in separating C1-fluoranthenes and
C1-pyrenes (four peaks should be observed in the
m/z
216
chromatogram), while complete resolution was observed
for 3-methylchrysene and 6-methylchrysene in both
methods. All four methylphenanthrenes are visible and
well separated from the 2-methylanthracene signal, in
contrast to the GC-MS separation where a coelution of the
two groups is observed. These results indicate that
isobaric-alkylated PAHs can be partially resolved using
single-column liquid chromatography.
Figure 2. Comparison of peaks of PAHs contained in the Standard Reference Material
1491a, obtained by GC-MS analysis (1/10 dilution in hexane, top) and by SPE-LC-MS/
MS analysis (1/27,500 serial dilution in seawater, bottom ). Reference material listed
compounds: C1-naphthalenes (1-methyl, 2-methyl); C2-naphthalenes (1,2-dimethyl,
1,6-dimethyl, 2,6-dimethyl); C1-phenanthrenes (1-methyl, 2-methyl, 3-methyl, 9-methyl);
C1-anthracenes (2-methyl); C1-fluoranthenes (1-methyl, 3-methyl); C1-pyrenes (1-methyl,
4-methyl); C1-chrysenes (3-methyl, 6 methyl). Standard Reference Material 1491a also
contains one C2- phenanthrene (1,7-dimethyl, not shown)